Genetic loop keeps cellular clocks on time
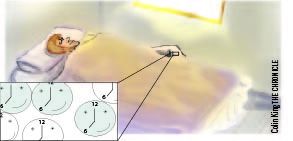
September 22, 2014
The human body’s circadian rhythm, synced with the 24 hour day, regulates almost every physiological process. New research has illuminated the interaction between four genes that control this circadian clockwork within each of the body’s trillions of cells.
Every cell houses four genes— Cryptochrome, Period, CLOCK and BMAL1—that keep time for the numerous biological components that depend on circadian rhythm. The influence that these genes have on one another determines how we cycle through the day.
“We’ve been aware of these four proteins since 1998,” said Dr. Aziz Sancar, the senior author of the study and professor of biochemistry and biophysics at the University of North Carolina. “The most obvious function they control is the sleep/wake cycle, or the activity/rest cycle. But blood, temperature, heart rate—you name it—they are all controlled by the clock.”
The cycle is a push-and-pull between these four genes, Sancar said. CLOCK and BMAL1 are expressed as proteins from within the nuclei of cells, instructing them to go about their business at the start of a circadian cycle approximately every 24 hours. The Period and Cryptochrome genes express outside of the nuclei of those cells, building protein complexes that reach a critical mass and re-enter the nuclei to bind to the CLOCK and BMAL1 proteins. This binding inhibits the expression of proteins that begin the clock’s cycle before the Cryptochrome and Period proteins themselves degrade, allowing the cycle to begin again.
“[What’s going on in the cell] is a negative feedback loop, so there’s no starting or stopping it,” said Jay Dunlap, professor of genetics at the Geisel School of Medicine at Dartmouth College. “It’s a cycle you can pick up at any time.”
Over the course of the cycle, Period and Cryptochrome proteins build up and re-enter the nucleus of the cell, binding to CLOCK and BMAL1 proteins to inhibit their activity. This signals the decrease of protein expression for the CLOCK and BMAL1, triggering the end of the cycle. Eventually these Period and Cryptochrome proteins break down and allow for the CLOCK and BMAL1 genes to begin expressing themselves within a cell again, signaling the beginning of a new day. The research focused on determining exactly how these four genes regulate one another and interact to influence cellular circadian cycles.
The team from UNC was able to modify cells so that instead of containing the four genes that relate to keeping time with the 24-hour cycle, they only contained the two that express the proteins responsible for starting the clock at the onset of the day: CLOCK and BMAL1. When Period was added back into the cells, it did not influence the behavior of the activator genes, but when Cryptochrome was added back into the modified cells without its Period counterpart, it completely deactivated CLOCK and BMAL1 without degrading. When Period was then added to the cells containing the other three genes, with Cryptochrome indefinitely inhibiting the wake-up call from CLOCK and BMAL1, Cryptochrome degraded, allowing for the circadian clock cycle to take place.
Dunlap said a delay in the negative feedback loop like the kind induced in the modified cells would cause an oscillation. This explains the positive/negative tug-of-war between the two sets of genes. CLOCK and BMAL1 drive the expression of Period and Cryptochrome, which eventually shut down the first two activator proteins, forming the core of the clock.
“The understanding of complex phenomena like the circadian clock begins with the identification of its components,” Dunlap said. “The understanding of knowing how a phenomenon works goes from knowing what the components are to understanding exactly what the components do.”
According to Dr. David K. Welsh, associate professor in the department of psychiatry at the University of California San Diego School of Medicine, there has been tremendous progress in understanding how this circadian clock works in recent decades. Researchers used to think there was only one place—a small area in the brain above where the optic nerves cross—that acted as a “master clock.” The recognition of a molecular mechanism for regulation of circadian rhythm has allowed for better understanding of how it regulates processes other than sleep, such as the balancing of nutrients, body temperature and weight regulation.
“When people have circadian clock disorders where you have a mutation in one of these regulatory genes, the primary complaint that they’ll usually initially seek medical attention for is disrupted sleep,” Welsh said.
Sleep, the most prominent aspect of physiology that varies throughout the course of the day, can be affected by a mismatch between the circadian clock and the surrounding environment. Genetic mutations can influence this disconnect as well as external factors like shift work or jet lag playing a role in the body’s ability to sync with the rhythm provided by trillions of cells measuring time in a 24 hour cycle.
Sancar said a better understanding of the clock’s genetic mechanism could have implications for an array of ailments that affect circadian rhythms, sleep, DNA repair and memory, among others.
“Unless you know how these proteins function exactly, the inhibitors would be non-specific,” Sancar said. “We hope that our finding will help to develop specific compounds for controlling the clock.”